Potential mechanisms for osteopathic manipulative treatment to alleviate migraine-like pain in female rats
- 1Department of Biomedical and Anatomical Sciences, New York Institute of Technology College of Osteopathic Medicine at Arkansas State University, Jonesboro, AR, United States
- 2Department of Osteopathic Manipulative Medicine, New York Institute of Technology College of Osteopathic Medicine at Arkansas State University, Jonesboro, AR, United States
Introduction: Migraines are the leading cause of disability in the United States, and the use of non-pharmaceutical treatments like osteopathic manipulative treatment (OMT) has shown promise. Despite its potential, the lack of mechanistic understanding has hindered widespread adoption. This study aims to investigate the efficacy of OMT in treating acute migraines and unravel its underlying mechanisms of action.
Methods: Female rats were subjected to a “two-hit” approach to induce migraine-like pain. This involved bilateral injections of Complete Freund's Adjuvant (CFA) into the trapezius muscle (1st hit) followed by exposure to Umbellulone, a human migraine trigger, on Day 6 post-CFA (2nd hit). Soft tissue and articulatory techniques were applied to the cervical region for acute abortive or repeated prophylactic treatment. Cutaneous allodynia and trigeminal system activation were assessed through behavioral tests and immunohistochemical staining.
Results: Following Umbellulone inhalation, CFA-primed rats exhibited periorbital and hind paw allodynia. Immediate application of OMT after Umbellulone inhalation as an abortive treatment partially alleviated cutaneous allodynia. With OMT applied thrice as a prophylactic measure, complete suppression of tactile hypersensitivity was observed. Prophylactic OMT also prevented the increase of c-fos signals in the trigeminal nucleus caudalis and the elevation of calcitonin gene-related peptide expression in trigeminal ganglia induced by CFA and Umbellulone exposure at 2 h post-inhalation.
Discussion: These findings provide mechanistic insights into OMT's migraine-relief potential and underscore its viability as a non-pharmacological avenue for managing migraines.
Introduction
Migraine, characterized by recurrent unilateral throbbing cephalic pain along with hypersensitivity to external stimuli, poses a significant debilitating challenge (1, 2). While the precise triggers for migraine attacks remain elusive, the activation of the trigeminovascular system stands as a pivotal factor in pain development (1, 3). Trigeminal activation has been recognized as a predictive indicator for migraine onset among sufferers (4). This activation leads to the release of excitatory neurotransmitters, especially calcitonin gene-related peptide (CGRP) from dural afferent terminals (5). Substantial evidence now supports a crucial role of CGRP in the pathophysiology of migraine (6, 7). Release of CGRP from nociceptors innervating the cranial meninges is thought to contribute to neurogenic vasodilation and to promote sensitization and activation of these fibers (7, 8). Sustained activation of peripheral nociceptors can elicit sensitization of the second-order neurons of the trigeminal nucleus caudalis (TNC) (3, 4) resulting in enhanced nociceptive inputs to higher brain centers including the thalamus, hypothalamus and cortical sites, collectively manifesting as migraine pain (4, 9).
Current migraine treatments, encompassing triptans, nonsteroidal anti-inflammatory drugs (NSAIDs) and CGRP antibodies/antagonists, yield response rates of approximately 50% (10–15). Due to limited efficacy or adverse effects, many migraine patients seek complementary approaches such as nutrition/diet adjustments, exercise, traditional Chinese medicine, and manual treatments like osteopathic manipulative treatment (OMT) (16–20). These options are cost-effective, well-tolerated, and generally entail minimal side effects. Nevertheless, the substantiation of their effectiveness, determined through double-blinded clinical trials, remains generally insufficient.
OMT is a form of manual treatment that targets the musculoskeletal system and emphasizes the intricate interplay between bodily structure and function. Involving soft tissue mobilization, joint manipulation, myofascial release, and other approaches, OMT aims to enhance blood circulation, diminish muscle tension, and foster the body's innate self-healing mechanisms. Although some reports suggest that OMT effectively reduces the frequency, intensity, and duration of migraine headaches and improve patients' quality of life (16, 19–21), the evidence supporting its efficacy for migraines remains inconclusive or of limited quality (20). Elucidating the potential mechanisms underpinning OMT's effects would substantially bolster the acceptance of this treatment strategy and promote evidence-based medical practice.
While migraines commonly associate with the ophthalmic division (V1) of the trigeminal nerve, over two-thirds of migraine patients also experience neck pain during migraine episodes or in the prodrome/postdrome phases, aligned with the receptive field of the greater occipital nerve (GON) originating from the C2 spinal nerve root (22–26). It has been suggested that some of the trigeminal afferents terminate at the C2 spinal segment (27–29). The anatomical and functional convergence of trigeminal and upper cervical afferent input at the trigeminal nucleus caudalis (TNC) might contribute to the concurrent head and neck pain (30). Furthermore, the functional convergence of sensorimotor fibers in the spinal accessory nerve (CN XI) and upper cervical nerve roots, along with the descending tract of the trigeminal nerve, may underlie the referral of cervical pain to the head (31). Nociceptive inputs from cervical muscles like the splenius capitis, upper trapezius, and sternocleidomastoid can sensitize the TNC, potentially intensifying cephalic pain (23, 30, 32). Massaging over the GON has been shown to reduce the intensity of migraine pain (33).
In this study, we developed a new migraine model built upon the established models characterized by the Durham (34) and Porreca (35) groups. This “two hit” model entails priming animals with an immunogenic inflammatory agent, Complete Freund Adjuvant (CFA), injected bilaterally into the trapezius muscles at a low dose to induce mild cervical inflammation and establish a state of “latent sensitization” (1st hit). By Day 5 post-CFA injection, the animals display normal sensory thresholds at the periorbital region. However, upon exposure to umbellulone (2nd hit), a major volatile molecule emitted by the “heacache tree” Umbellularia californica, cutaneous allodynia and activation of the TNC developed. Umbellulone, identified as a transient receptor potential ankyrin type 1 (TRPA1) agonist, interacts with this ion channel highly permeable to sodium and calcium ions (36, 37). TRPA1 receptors are primarily expressed in slowly conducting primary afferent neurons (38, 39), which mediate nociceptive and cold receptive functions (40). Activation of TRPA1 sensitizes meningeal afferents, potentially facilitating headache generation (41, 42).
Utilizing this new migraine model, we investigated the effects of OMT on migraine-like pain behavior and pathophysiology. Drawing from empirically employed human soft tissue (43) and articulatory (44) techniques by osteopathic practitioners, we adapted an OMT protocol for rats. This approach involved direct passive manipulation along the neck and upper torso regions to address migraines and cervicogenic headaches. We administered OMT to the neck muscles and cervical vertebra of the rats before or after the migraine trigger and assessed the efficacy of acute or preventative OMT in treating migraines in this model. Additionally, we explored TNC activation and CGRP expression in trigeminal ganglia (TG) tissues, aiming to glean mechanistic insights into how OMT relieves migraines.
Methods
Animals
Adult female Sprague-Dawley rats with an initial weight of 175–200 g (Charles River) were used for the experiments. The rats were housed in groups of 4 per cage on a 12 h light-dark cycle (lights on at 8 AM). They have ad libitum access to standard autoclaved lab chow and water. The allocation of animals to different treatment groups was randomized ensuring impartial distribution. All experiments were performed by experimenters blinded to the treatment groups and the blinding codes were not revealed until after the data analysis was completed. Group size for each experiment was determined by power analysis (see the Statistical Analysis below).
Rat migraine model
The rats were anesthetized with 2% isoflurane at 1 L/min. Trigeminal sensitization was induced by injecting CFA (Sigma-Aldrich, St. Louis, MO) diluted 1:1 in 0.9% sterile saline. The injections were distributed across ten different sites in the trapezius muscle between C1 and C4 vertebrae. Each side received 5 injections of 10 μl each, resulting in a bilateral total of 100 μl (50 μl per side). After the injections, the animals were allowed to recover in their home cages for 6 days. On the 6th day, rats were placed in a Plexiglas chamber (dimensions: 23 × 9.5 × 9 cm height) connected to an O2 tank for exposure to umbellulone (Sigma-Aldrich, St. Louis, MO). Umbellulone was dissolved completely in DMSO and then diluted 20-fold with deionized water (DiH2O) to achieve a final concentration of 0.05 M immediately before use. A small cotton ball soaked with 50 μl of umbellulone was placed within a small tube positioned at the chamber entrance, allowing continuous delivery of umbellulone to the chamber at a flow rate of 2 L/min for 30 min. Following the umbellulone exposure, rats were returned to their home cages. The sham treatment group received a vehicle solution comprising 5% DMSO and 95% DiH2O for 30 min using the same delivery method.
Behavioral testing for tactile hypersensitivity
All behavioral evaluations were carried out between 9 AM and 3 PM (35). Prior to testing, rats were acclimated to the Plexiglas testing chambers (33 × 17 × 20 cm height) containing a wired mesh floor over a period of 1 h daily for 5 consecutive days. Mechanical thresholds were determined in response to a series of calibrated von Frey (VF) filaments (Stoelting, Wood Dale, IL) applied in increasing force to the cutaneous tissue. The cutoff threshold was set at 8 g for the periorbital region and 15 g for the hind paw region. Baseline thresholds were determined prior to any experimental procedures. A positive response, indicated by head or paw withdrawal upon filament contact, was recorded. The up-down method was adopted, and withdrawal thresholds were calculated using the Dixon method (45). The experimental procedure is illustrated in Figure 1.
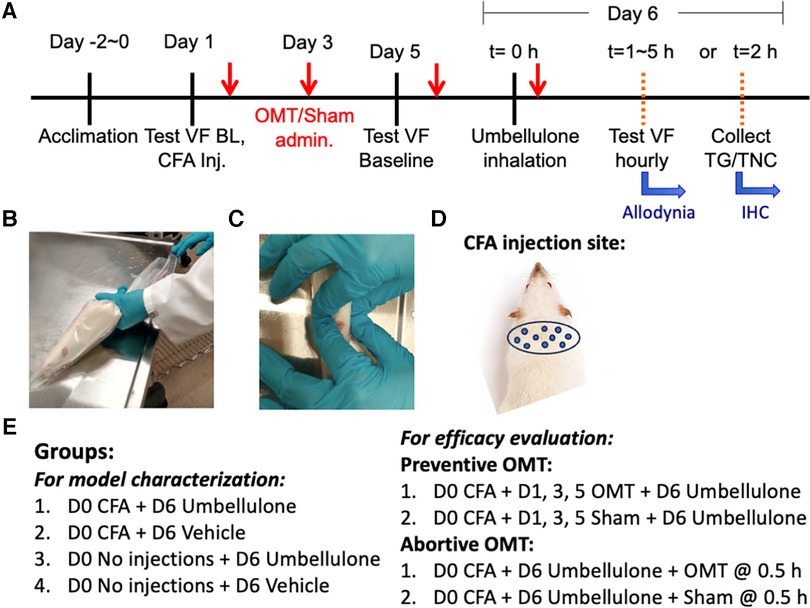
Figure 1. Experimental plan and application of OMT. Panel (A) illustrates the study design and experimental timeline. The animals were gently restrained inside the plastic decapicones while awake (B) OMT were applied to the neck region using 1 min soft tissue techniques followed by 1 min of articulatory techniques, as depicted in Panel (C) Sham animals were only restrained for 2 min without any manipulations. The injection sites for CFA are illustrated in Panel (D) the experimental groups are listed in Panel (E).
Osteopathic manipulative treatment (OMT) procedure
Adapting human manipulations, we introduced a modified soft tissue technique involving bilateral lateral stretch and an articulatory technique applied to cervical vertebrae in rats (Figure 1). Rats were restrained in a decapicone on a treatment table. An experimenter positioned a finger on each side of the rat's neck. Traction was applied bilaterally to the trapezius muscle, moving up and down along the neck for 1 min to relax local tissues. Subsequently, the articulatory technique was applied by gently translating cervical vertebrae side-to-side to remove any restrictive barriers by guiding the joint through its full range of motion for an additional minute, focusing on the neck. Following these maneuvers, traction was released, and palpation ceased. The rat was then returned to its home cage. Dr. Regina Fleming, D.O., a practitioner experienced in similar techniques, developed this treatment regimen. The form and duration of the treatment was determined by Dr. Fleming based on the desired outcomes, primarily enhanced relaxation of the cervical muscles and an augmented translatory motion bilaterally in the cervical spine, assessed through her fingers during the preliminary studies.
Immunohistochemical staining
Immunostaining was conducted following previously described methods (46). Briefly, at 2 h post-umbellulone inhalation, the animals were deeply anesthetized with ketamine/xylazine (100/12 mg/kg, i.p.) and subjected to transcardial perfusion with 4% paraformaldehyde. TG and TNC tissues were harvested and cryoprotected with 30% sucrose. The tissues were then dissected into thin slices of 15 μm (TG) and 30 μm (TNC) at −20°C using a cryostat. Slides from each experimental condition were washed in phosphate-buffered saline (PBS) 3 times for 3 min each, followed by blocking using a PBS solution containing 0.2% Tween20, 1% bovine serum albumin, and 2% glycine for 2 h at room temperature. Primary antibodies, diluted in PBS containing 3% goat serum and 0.05% Triton X-100, were incubated with the tissues over night at 4°C. Alexa Fluor secondary antibodies, diluted in PBS, were incubated with tissues at room temperature for 1 h. The VECTASHIELD medium was employed for tissue mounting, and fluorescence microscopy was performed using the BioTek Cytation-5 Bioimager/Plate Reader (Winooski, VT, USA). Gen-5 analysis software was utilized to quantify the number of cells expressing the specific markers using an unbiased manner based on fluorescence. To ensure consistent comparison for quantifications, tissues from all groups were processed in parallel to account for batch-to-batch variations. Within each batch, the exposure time, region of interest and quantification threshold were set by an experimenter blinded to the grouping information based on the entire batch of samples. Cell counts were obtained from 3 independent experiments, and 4–6 standardized regions of interest encompassing the TG or TNC were analyzed for each tissue sample. Mean values were determined for each animal, and data were reported as average cell counts per group with individual values indicated by the scatter plots. The detailed information of the antibodies is listed in Table 1.
Statistical analysis
All statistical analyses were performed using GraphPad Prism 9 software. Sample size was determined using G*Power3.1 based on historical data, with a set power (1-β) of 0.95 and an α error of 0.05. Data were presented as mean ± SEM. One-way ANOVA followed by Tukey's multiple comparison post hoc analysis or Two-way ANOVA followed by Dunnett's multiple comparison post hoc analysis was employed as appropriate. Statistical significance was set at P ≤ 0.05.
Results
Umbellulone induced tactile allodynia in CFA-primed rats
In the novel rat migraine model induced by umbellulone inhalation in CFA-primed female SD rats (Figure 2), we assessed the tactile hypersensitivity in the periorbital and hind paw regions. Initial baseline periorbital and hind paw withdrawal thresholds were 8.0 ± 0.0 & 15.0 ± 0.0 g, respectively, in naïve rats. CFA (5 injections/side, 10 μl each, i.m.) was injected bilaterally into the upper trapezius muscle under brief isoflurane anesthesia. Tactile threshold was evaluated on Days 3 and 5 post-CFA. Periorbital allodynia was absent at both time points with the tactile threshold remained at 8.0 ± 0.0 g. The hind paw threshold did not decrease significantly, either, compared to the baseline (9.6 ± 1.2 and 10.2 ± 0.9 g at Day 3 and 5 post-CFA, respectively, N = 8/group, P > 0.05).
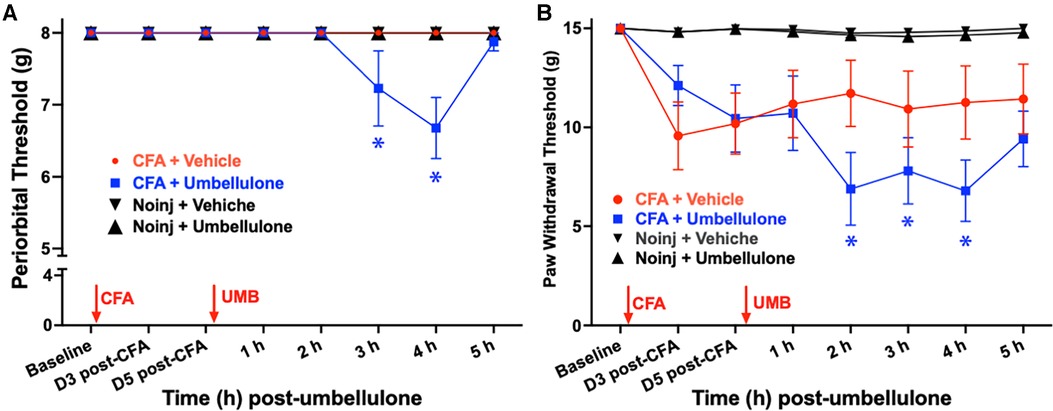
Figure 2. Effects of CFA priming and umbellulone inhalation on periorbial and hind paw tactile thresholds. Rats received bilateral injections of CFA (1:1 diluted with saline, 10 × 10 μl) into the trapezius muscle. Periorbital tactile threshold was measured at baseline (BL), as well as on Days 3 and 5 post-CFA, and hourly for 5 h on Day 6 after inhalation of umbellulone (50 mM, 50 μl). Cephalic allodynia developed at 2 and 3 h post-umbellulone inhalation in CFA-primed animals, while hind paw allodynia was observed at 2, 3 and 4 h post-umbellulone inhalation. Statistical analysis was performed using two-way ANOVA post hoc Dunnett's multiple comparison test. *P < 0.05 compared to the BL within the same group. N = 8/group.
On day 6, rats were exposed to umbellulone (50 mM, 50 μl) via inhalation for 30 min in a Plexiglas chamber using 2% O2. After umbellulone exposure, rats were placed in the VF chambers, and cutaneous tactile thresholds were assessed hourly for 5 h afterwards. CFA-primed animals exhibited significant periorbital and hind paw allodynia following umbellulone administration. The average periorbital threshold was reduced to 7.2 ± 0.5 and 6.6 ± 0.4 g at 3 & 4 h post-umbellulone, respectively, in the CFA + Umbellulone group (P < 0.05 compared to the corresponding baseline thresholds of each group or at the same time point of No Injection + Umbellulone group). In contrast, no change was observed in the CFA + Vehicle or No injection + Umbellulone groups. Similarly, the average hind paw threshold was reduced to 6.9 ± 1.8, 7.8 ± 1.7 and 6.8 ± 1.6 g at 2, 3 & 4 h post-umbellulone, respectively in CFA + Umbellulone group (P < 0.05 compared to the corresponding baseline thresholds of each group or at the same time point of No Injection + Umbellulone groups). The animals in the CFA + Vehicle or No Injection + Umbellulone groups did not show significant reduction of the hind paw tactile threshold from the baseline at any of the measured time points. N = 8/group.
These findings suggest that mild inflammation induced by CFA in the trapezius muscle created a “latent sensitization” state in naïve rats, rendering them more susceptible to the migraine trigger—umbellulone. It is important to note that the vehicle of umbellulone (5:95 DMSO:H2O) did not elicit noticeable effects in CFA-treated rats. Thus, both CFA priming and TRPA1 agonist umbellulone were necessary for inducing cephalic allodynia in naïve rats. This “double-hit” strategy replicates the increased susceptibility to sub-threshold triggers observed in migraineurs, where the control group remains asymptomatic in the absence of migraine triggers.
OMT acutely abolished the development of cutaneous allodynia
After establishing the migraine model, we examined the acute effects of OMT as an abortive treatment for migraine-like pain (Figure 3). Animals received the two hits (CFA and umbellulone) as described above. Immediately after umbellulone inhalation, a single session of OMT (1 min each of soft tissue and articulatory techniques applied to the neck region) or sham (confined in plastic cones for 2 min) was administered. As anticipated, umbellulone administration induced periorbital and hind paw allodynia, shown by the significant reduction of the tactile threshold at 3 h post-sham treatment (7.3 ± 0.2 & 8.3 ± 0.7 g for periorbital and hind paw threshold, respectively; P < 0.05 vs. baseline). However, a single application of OMT as an abortive treatment reduced the allodynia in CFA-primed rats. The periorbital tactile threshold was maintained at the baseline level and the hind paw threshold was partially restored to 10.5 ± 0.5 g at 3 h post-Umbellulone and OMT administration (P > 0.05 vs. baseline). N = 8/group.
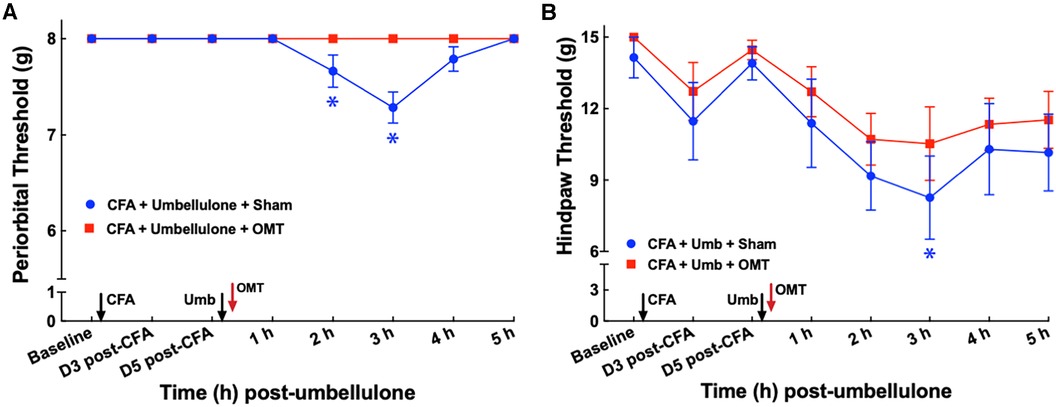
Figure 3. Blockade of periorbital (A) and hind paw (B) tactile allodynia by OMT as an abortive treatment. Animals were treated with CFA (1:1 dilution with saline) and umbellulone (50 mM, 50 μl) or its vehicle (5% DMSO/95% H2O). OMT was applied immediately after umbellulone inhalation in awake animals. Umbellulone significantly decreased the tactile threshold selectively in CFA-primed rats at 2 and 3 h post-dose. OMT inhibited the development of periorbital and hind paw (to a lesser extent) allodynia induced by umbellulone in CFA-primed rats. Statistical analysis was performed using two-way ANOVA post hoc Dunnett's multiple comparison test. *P < 0.05 compared to the baseline (BL) within the same group. N = 8/group.
Preventive application of OMT blocked the development of cutaneous allodynia
To examine the effect of OMT as a preventive treatment for migraine-like pain, we administered 3 episodes of OMT on Days 1, 3 & 5 post-CFA in animals receiving CFA and Umbellulone treatment (Figure 4). Following umbellulone inhalation, sham-treated animals developed cutaneous allodynia as expected, shown by the significant reduction of the periorbital and hind paw threshold to 6.7 ± 0.8 and 7.7 ± 0.2 g, respectively (P < 0.05 vs. baseline). However, animals receiving 3 preventive applications of OMT after CFA and prior to umbellulone administration exhibited a complete blockade of cutaneous allodynia development throughout the entire time course. At 3 h post-umbellulone, their periorbital and hind paw tactile threshold remained at 7.7 ± 0.2 & 12.3 ± 0.2, respectively (P > 0.05 compared to the baseline). This batch of animals also exhibited significant hind paw tactile hypersensitivity at Days 3 & 5 post-CFA in sham-treated animals, which was also blocked by the OMT prophylactic treatment. At Day 3 post-CFA, the average hind paw threshold was 8.9 ± 0.4 g (P < 0.05 vs. baseline) and 12.4 ± 0.3 g (P > 0.05 vs. baseline) for sham and OMT-treated animals, respectively (N = 8/group).
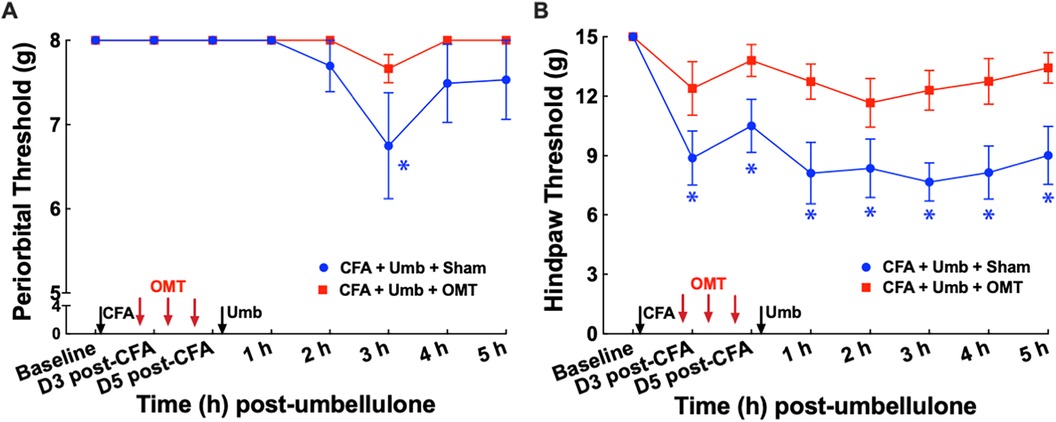
Figure 4. Blockade of periorbital (A) and hind paw (B) tactile allodynia by preventive OMT. Animals were treated with CFA (1:1 dilution with saline) and umbellulone (50 mM, 50 μl) or its vehicle (5% DMSO/95% H2O). OMT was applied on Days 1, 3 and 5 after CFA injections prior to umbellulone inhalation as a preventive treatment. Umbellulone significantly reduced periorbital and hind paw thresholds selectively in CFA-primed rats. However, application of OMT completely prevented the development of periorbital and hind paw allodynia induced by umbellulone in CFA-primed rats. Statistical analysis was performed using two-way ANOVA post hoc Dunnett's multiple comparison test. *P < 0.05 compared to the baseline (BL) within the same group. N = 8/group.
OMT blocked the activation of TNC neurons
To investigate the underlying mechanisms of OMT in alleviating migraine-like pain, we assessed its effects on the activation of the trigeminal system, including peripheral neurons in the TG and central neurons in the brainstem TNC. Animals received CFA and umbellulone, along with 3 applications of preventive OMT on Days 1, 3, & 5 post-CFA, as described above. At 2 h post-umbellulone inhalation, the animals were sacrificed via transcardial perfusion of the fixatives, and the TG and TNC tissues were processed immunohistochemically. We quantified the expression of c-fos, an immediate early response gene, to assess neuronal activation in the TNC. The number of c-fos+ cells was quantified from 6 independent slices of the TNC tissues for each animal (Figure 5). The expression of c-fos more than doubled after umbellulone inhalation in CFA-primed, sham-treated rats (CFA + Umbellulone + Sham, 1,728 ± 249) compared to the rats without priming (No injection + Umbellulone + Sham, 616 ± 253, P < 0.05) or primed without umbellulone inhalation (CFA + Vehicle + Sham, 574 ± 225, P < 0.05). However, the application of preventive OMT completely blocked this increase (CFA + Umbellulone + OMT, 492 ± 160, P < 0.05 vs. CFA + Umbellulone + Sham group). N = 4–6/group with individual group size shown in the scatter plot in Figure 5I.
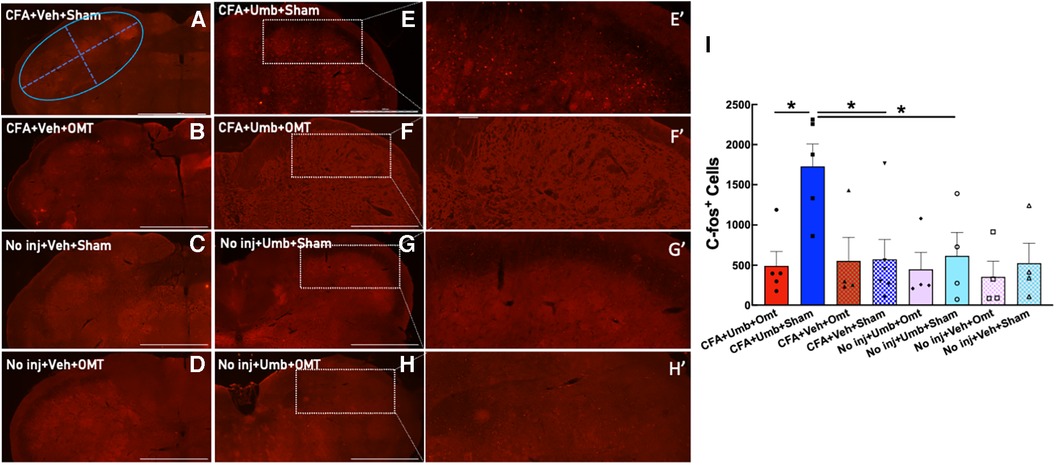
Figure 5. Blockade of trigeminal nucleus caudalis (TNC) activation by preventive OMT. Animals were treated with CFA (1:1 dilution with saline) and umbellulone (50 mM, 50 μl) or its vehicle (5% DMSO/95% H2O). OMT was applied on Days 1, 3 and 5 after CFA injections prior to umbellulone inhalation as a preventive treatment. Umbellulone significantly increased the expression of c-fos in TNC at 2 h after umbellulone treatment selectively in CFA-primed rats. However, application of OMT successfully abolished this enhancement. E’,F’,G’,H’ represent amplified images of the rectangle regions in E,F,G,H. The number of c-fos positive cells in all images was quantified automatically via Cytation 5 using the same pre-set Region of Interest (ROI) illustrated by the blue oval in Panel (A) with the long diameter of 2,400 μm and the short diameter of 1,000 μm. The bar graph (I) illustrates quantified data with individual data points shown in the scatter lot. Each data point represents the average number of c-fos+ cells of 6 independent (non-adjacent) TNC slices from the same animal. Statistical analysis was performed using one-way ANOVA post hoc Tukey's multiple comparison test. *P < 0.05 compared to the corresponding group. N = 4–6/group. Scale bar: 1,000 μm.
OMT suppressed the elevation of CGRP expression in TG neurons
CGRP has been identified to play a pivotal role in migraine pathophysiology. We measured CGRP expression in peripheral TG neurons at 2 h after umbellulone exposure. Animals were treated as described above, and the TG tissues were collected and processed for immunohistochemistry. The number of CGRP+ cells was quantified from 4 independent slices of the TG tissues for each animal (Figure 6). The results showed that CGRP expression was significantly increased in the CFA + Umbellulone + Sham group (111 ± 7) compared to the CFA-primed, but without umbellulone trigger group (CFA + Vehicle + Sham, 36 ± 5, P < 0.01) or the group without priming but with umbellulone trigger (No injection + Umbellulone + Sham, 48 ± 4, P < 0.01). However, the application of OMT on Days 1, 3 and 5 post-CFA completely blocked this increase (CFA + Umbellulone + OMT, 41 ± 4, P < 0.01 vs. CFA + Umbellulone + Sham group). N = 4–8/group with individual group size shown in the scatter plot in Figure 6I.
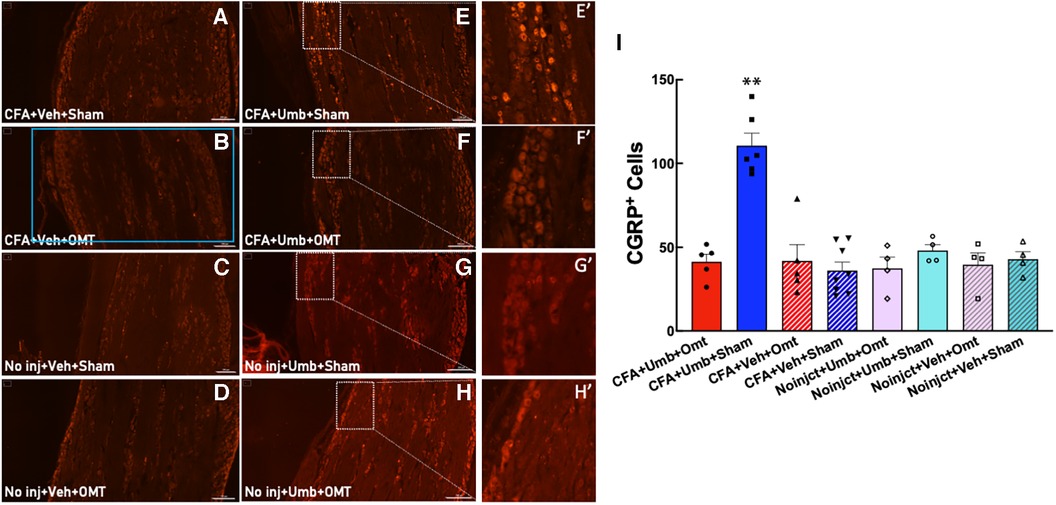
Figure 6. Blockade of the CGRP expression in trigeminal ganglia (TG) by preventive OMT. Animals were treated with CFA (1:1 dilution with saline) and umbellulone (50 mM, 50 μl) or its vehicle (5% DMSO/95% H2O). OMT was applied on Days 1, 3 and 5 after CFA injections prior to umbellulone inhalation as a preventive treatment. Umbellulone significantly increased the expression of CGRP in TG at 2 h after umbellulone treatment selectively in CFA-primed rats. However, application of OMT successfully prevented this enhancement. E’,F’,G’,H’ represent amplified images of the rectangle regions in E,F,G,H. The number of CGRP positive cells in all images was quantified automatically via Cytation 5 using the same pre-set Region of Interest (ROI) illustrated by the blue rectangle in Panel (B) with the sides of 2,200 × 1,200 μm. The bar graph (I) illustrates quantified data with individual data points shown in the scatter lot. Each data point represents the average number of CGRP+ cells of 4 independent (non-adjacent) TG slices from the same animal. Statistical analysis was performed using one-way ANOVA post hoc Tukey's multiple comparison test. *P < 0.05 compared to any other group. N = 4–8/group. Scale bar: 200 μm.
Discussion
OMT presents a significant non-pharmacological strategy to treat migraine pain. However, despite its efficacy, the precise mechanisms through which OMT exerts its effects have remained unexplored, hampering its widespread utilization. In this study, we created a new rodent model to explore the impact of OMT on both behavior manifestations and physiological mechanisms underpinning migraine pathology. Our findings offer compelling evidence that multiple preventive applications of OMT in sensitized rats effectively blocked the development of cutaneous tactile allodynia—a hallmark of migraine pain. This effect appears to be mediated via the modulation of the trigeminal system, involving the inhibition of CGRP overexpression in primary sensory neurons and suppression of secondary order neuron activation within the brainstem. Interestingly, OMT applied after the umbellulone trigger partially diminished the development of allodynia, indicating that early intervention with OMT may be more effective, consistent with clinical observations (47).
Mounting evidence supports the crucial role of trigeminocervical complex activation in migraine pathophysiology. There have been multiple animal models of migraine to recapitulate different aspects of human migraine pain. The initial rat model of migraine directly activates the trigeminal neurons or the meninges by electrical stimulation or injection of inflammatory mediators (48–52). These models elicit activation of the trigeminal system to produce robust cephalic and extracephalic allodynia. However, they are invasive and produce injuries to the skull, which is absent in primary migraine headache. Subsequently, several non-invasive migraine models were created to mimic this key feature of migraine (6, 34, 53). Our novel migraine model exhibited that the TRPA1 agonist umbellulone selectively induced cephalic and extracephalic allodynia in CFA-primed animals, corroborating the observations from the Durham (34) and Porreca (35) research groups. Diverging from the Durham model that employed California bay leaf extract as the migraine trigger, we utilized pure umbellulone, as adopted by the Porreca group, to ensure precise control of the trigger dosage and circumvent inconsistencies associated with different batches of plant materials. By maintaining the umbellulone dose at a subthreshold level, we successfully avoided the induction of migraine-like pain in naïve (non-primed) animals. Additionally, we introduced mild neck inflammation through intratrapezius injections of CFA based on the Durham model to mimic the conditions often observed in patients with cervical muscle fatigue, which can trigger migraine attacks. Critically, animals receiving both CFA and umbellulone exhibited similar pathophysiological changes in the trigeminal system, including CGRP overexpression in the TG and activation of second order neurons in the TNC. Notably, these changes were effectively normalized by the prophylactic administration of OMT.
Clinically, two randomized, sham-controlled clinical trials encompassing a total of 148 chronic migraine patients have examined the therapeutic effects of OMT on migraine headaches (43, 54). Both studies reported superior efficacy of OMT over sham treatments, with multiple OMT sessions significantly reducing the frequency of migraine days per month. The treatment protocols in these trials encompassed various techniques such as myofascial release, balanced ligamentous tension, balanced membranous tension, and cranial-sacrum techniques such as suboccipital release. Drawing inspiration from these clinical maneuvers, we designed the current OMT protocol employing soft tissue and articulatory techniques to emulate clinical practice.
Our manipulations targeted the four muscles constituting the posterior suboccipital triangle: rectus capitis posterior major (RCPMa), rectus capitis posterior minor (RCPmi), obliquus capitis inferior (OCI), and obliquus capitis superior (OCS). These muscles are innervated by the dorsal ramus of C1, situated beneath the upper trapezius (innervated by spinal accessory nerve CN XI, C3 and C4) and overlay the deeper neck and throat myofascial tissues innervated by cervical spinal nerves C1–C4. It is worth noting that RCPMa and RCPmi have a connective bridge attachment to the dura mater (55, 56), establishing a potential link between tension reduction in the suboccipital triangle and headache alleviation. This tension reduction likely promotes myofascial relaxation and improved vascular flow dynamics, along with the inhibition of the first cervical nerve (C1), the greater occipital nerve (a medial branch of dorsal ramus of C2), and the lesser occipital nerve (dorsal ramus of C3). Given their heightened density of muscle spindles relative to larger muscles, OCS and RCPMa are particularly sensitive to stretch and exhibit strain with light force application (57). This phenomenon is observed across different species, similar to the calming effect experienced by human infants when caressed at the base of the skull or when baby animals are carried by the nape of their necks (58–60). Therefore, the soft tissue and articulatory techniques employed in our study may elicit relaxation in rats that is analogous to the release of muscle tension observed in humans.
It is worth noting that the OMT was performed in awake animals under brief restraint instead of anesthesia. This was based on our preliminary data that isoflurane anesthesia might interfere with the development of the cephalic pain either during the priming phase or abortive treatment phase. Thus, we chose to briefly restrain the animals to perform OMT awake. The concerns of the potential restraint stress were mitigated by the lack of tactile hypersensitivity in the CFA + Umbellulone + Sham group for both abortive and preventive experiments. Indeed, much more intense restraint stress is required to induce migraine-like pain phenotype as shown by the Porreca group. They have reported using 1 h/day × 2 days of bright light stress to trigger migraine in drug-primed rats (61) or 2 h/day × 3 days of restraint stress to prime the mice so they are sensitive to umbellulone trigger (35). The stimulus intensity of the brief 2 min restriction in our experiments was far below the migraine-eliciting ones.
The underlying pathophysiological mechanisms for OMT to alleviate cephalic pain has not been elucidated previously. Our results revealed that recurrent prophylactic OMT applications effectively inhibited CGRP overexpression in primary sensory neurons within TG and the enhanced activation of secondary order neurons in TNC prompted by umbellulone in CFA-primed rats. CGRP and its receptors are known to be expressed in TG neurons of both rodents and humans with similar patterns (62–64). CGRP is expressed in about 50% TG neurons, primarily encompassing unmyelinated small to medium-sized neurons, particularly c-fibers responsible for transmitting pain sensation (62–64). Our results demonstrated significantly higher levels of CGRP expression in TG neurons selectively in the CFA + Umbellulone + Sham group, underscoring the pivotal role of CGRP in the development of migraine in this novel model. Similarly, the augmented activation of second order neurons in TNC following the priming and migraine trigger was also consistent with other rodent migraine models and human imaging studies (65, 66). The soft tissue and articulatory techniques adopted in our study exhibited remarkable efficacy to suppress both groups of neurons, potentially representing the core molecular mechanisms driving OMT's migraine-alleviating effect.
OMT is a highly individualized treatment approach, tailored to address the specific needs of each patient. Despite the variances in individual treatment plans, similar techniques have been used to treat different kinds of headaches with underlying pathophysiological changes. For instance, myofascial release has garnered considerable success in treating tension-type headaches (67). In our study, we focused on soft tissue and articulatory techniques and their effects in a specific migraine model. Further investigations are needed to determine if these techniques can exert similar effects in other migraine models, thereby broadening our understanding of their applicability across diverse migraine types.
Overall, our research provides invaluable insights into the mechanisms underpinning the therapeutic effects of OMT for migraine-like pain. By unveiling the impact of OMT on the trigeminal system, including its ability to modulate CGRP expression and neuronal activation, we contribute substantially to the scientific understanding of OMT's physiological basis in mitigating migraines. These findings further support the clinical adoption of OMT as an efficacious non-pharmacological intervention for alleviating migraine-associated pain.
Highlights
• Inhalation of umbellulone induced periorbital and hind paw tactile allodynia in rats sensitized with Complete Freund's Adjuvant (CFA).
• Osteopathic manipulative treatment (OMT) given as a preventive or abortive treatment blocked umbellulone-induced cutaneous allodynia.
• Preventive OMT applications curbed abnormal activation of trigeminal nucleus caudalis induced by umbellulone.
• OMT suppressed the elevation of calcitonin gene-related peptide (CGRP) expression in trigeminal ganglia induced by umbellulone inhalation.
Data availability statement
The original contributions presented in the study are included in the article/Supplementary Material, further inquiries can be directed to the corresponding author.
Ethics statement
All behavioral and immunohistochemical studies were conducted in compliance with the guidelines set by the National Institute of Health for laboratory animals and approved by the Institutional Animal Care and Use Committee (IACUC) of Arkansas State University.
Author contributions
KB: Data curation, Formal Analysis, Investigation, Writing – review & editing. ML: Data curation, Formal Analysis, Investigation, Writing – review & editing. YP: Data curation, Formal Analysis, Investigation, Writing – review & editing. BC: Data curation, Investigation, Writing – review & editing. KC: Investigation, Writing – review & editing. DF: Investigation, Writing – review & editing. JB-M: Investigation, Writing – review & editing. MS: Investigation, Writing – review & editing. HH: Investigation, Writing – review & editing. CG: Investigation, Writing – review & editing. RF: Conceptualization, Investigation, Writing – review & editing. JX: Conceptualization, Formal Analysis, Funding acquisition, Project administration, Supervision, Writing – original draft, Writing – review & editing.
Funding
The author(s) declare financial support was received for the research, authorship, and/or publication of this article.
This research is supported by the National Institutes of Health (NIH)/National Center for Complementary and Integrative Health (NCCIH) R15 grant (No. 1R15AT011097-01) awarded to JX.
Conflict of interest
The authors declare that the research was conducted in the absence of any commercial or financial relationships that could be construed as a potential conflict of interest.
Publisher's note
All claims expressed in this article are solely those of the authors and do not necessarily represent those of their affiliated organizations, or those of the publisher, the editors and the reviewers. Any product that may be evaluated in this article, or claim that may be made by its manufacturer, is not guaranteed or endorsed by the publisher.
References
1. Noseda R, Burstein R. Migraine pathophysiology: anatomy of the trigeminovascular pathway and associated neurological symptoms, cortical spreading depression, sensitization, and modulation of pain. Pain. (2013) 154(Suppl 1):S44–53. doi: 10.1016/j.pain.2013.07.021
3. Bernstein C, Burstein R. Sensitization of the trigeminovascular pathway: perspective and implications to migraine pathophysiology. J Clin Neurol. (2012) 8:89–99. doi: 10.3988/jcn.2012.8.2.89
4. Stankewitz A, Aderjan D, Eippert F, May A. Trigeminal nociceptive transmission in migraineurs predicts migraine attacks. J Neurosci. (2011) 31:1937–43. doi: 10.1523/JNEUROSCI.4496-10.2011
5. Edvinsson L. Calcitonin gene-related peptide (CGRP) is a key molecule released in acute migraine attacks-successful translation of basic science to clinical practice. J Intern Med. (2022) 292(4):575–86. doi: 10.1111/joim.13506
6. De Felice M, Ossipov MH, Wang R, Dussor G, Lai J, Meng ID, et al. Triptan-induced enhancement of neuronal nitric oxide synthase in trigeminal ganglion dural afferents underlies increased responsiveness to potential migraine triggers. Brain. (2010) 133:2475–88. doi: 10.1093/brain/awq159
7. Raddant AC, Russo AF. Calcitonin gene-related peptide in migraine: intersection of peripheral inflammation and central modulation. Expert Rev Mol Med. (2011) 13:e36. doi: 10.1017/S1462399411002067
8. Bigal ME, Walter S, Rapoport AM. Calcitonin gene-related peptide (CGRP) and migraine current understanding and state of development. Headache. (2013) 53:1230–44. doi: 10.1111/head.12179
9. Burstein R, Collins B, Jakubowski M. Defeating migraine pain with triptans: a race against the development of cutaneous allodynia. Ann Neurol. (2004) 55:19–26. doi: 10.1002/ana.10786
10. Croop R, Lipton RB, Kudrow D, Stock DA, Kamen L, Conway CM, et al. Oral rimegepant for preventive treatment of migraine: a phase 2/3, randomised, double-blind, placebo-controlled trial. Lancet. (2021) 397:51–60. doi: 10.1016/S0140-6736(20)32544-7
11. Edvinsson L, Haanes KA, Warfvinge K, Krause DN. CGRP As the target of new migraine therapies—successful translation from bench to clinic. Nat Rev Neurol. (2018) 14:338–50. doi: 10.1038/s41582-018-0003-1
12. Ferrari MD, Roon KI, Lipton RB, Goadsby PJ. Oral triptans (serotonin 5-HT(1B/1D) agonists) in acute migraine treatment: a meta-analysis of 53 trials. Lancet. (2001) 358:1668–75. doi: 10.1016/S0140-6736(01)06711-3
13. Kelley NE, Tepper DE. Rescue therapy for acute migraine, part 3: opioids, NSAIDs, steroids, and post-discharge medications. Headache. (2012) 52:467–82. doi: 10.1111/j.1526-4610.2012.02097.x
14. Nedd M, Garland S, Falk N, Wilk A. Ubrogepant: an oral calcitonin gene-related peptide (CGRP) receptor antagonist for abortive migraine treatment. Ann Pharmacother. (2022) 56(3):346–51. doi: 10.1177/10600280211023810
15. Pascual J, Mateos V, Roig C, Sanchez-Del-Rio M, Jimenez D. Marketed oral triptans in the acute treatment of migraine: a systematic review on efficacy and tolerability. Headache. (2007) 47:1152–68. doi: 10.1111/j.1526-4610.2007.00849.x
16. Cerritelli F, Lacorte E, Ruffini N, Vanacore N. Osteopathy for primary headache patients: a systematic review. J Pain Res. (2017) 10:601–11. doi: 10.2147/JPR.S130501
17. Millstine D, Chen CY, Bauer B. Complementary and integrative medicine in the management of headache. Br Med J. (2017) 357:j1805. doi: 10.1136/bmj.j1805
18. Moore C, Adams J, Leaver A, Lauche R, Sibbritt D. The treatment of migraine patients within chiropractic: analysis of a nationally representative survey of 1869 chiropractors. BMC Complement Altern Med. (2017) 17:519. doi: 10.1186/s12906-017-2026-3
19. Holland S, Silberstein SD, Freitag F, Dodick DW, Argoff C, Ashman E. Evidence-based guideline update: nSAIDs and other complementary treatments for episodic migraine prevention in adults: report of the quality standards subcommittee of the American academy of neurology and the American headache society. Neurology. (2012) 78:1346–53. doi: 10.1212/WNL.0b013e3182535d0c
20. Smith MS, Olivas J, Smith K. Manipulative therapies: what works. Am Fam Physician. (2019) 99:248–52.30763049
21. Wells RE, Beuthin J, Granetzke L. Complementary and integrative medicine for episodic migraine: an update of evidence from the last 3 years. Curr Pain Headache Rep. (2019) 23:10. doi: 10.1007/s11916-019-0750-8
22. Blau JN, MacGregor EA. Migraine and the neck. Headache. (1994) 34:88–90. doi: 10.1111/j.1526-4610.1994.hed3402088.x
23. Florencio LL, Ferracini GN, Chaves TC, Palacios-Cena M, Ordas-Bandera C, Speciali JG, et al. Active trigger points in the cervical musculature determine the altered activation of superficial neck and extensor muscles in women with migraine. Clin J Pain. (2017) 33:238–45. doi: 10.1097/AJP.0000000000000390
24. Kaniecki RG. Migraine and tension-type headache: an assessment of challenges in diagnosis. Neurology. (2002) 58:S15–20. doi: 10.1212/wnl.58.9_suppl_6.s15
25. Tfelt-Hansen P, Lous I, Olesen J. Prevalence and significance of muscle tenderness during common migraine attacks. Headache. (1981) 21:49–54. doi: 10.1111/j.1526-4610.1981.hed2102049.x
26. Waelkens J. Warning symptoms in migraine: characteristics and therapeutic implications. Cephalalgia. (1985) 5:223–8. doi: 10.1046/j.1468-2982.1985.0504223.x
27. Angus-Leppan H, Lambert GA, Boers P, Zagami AS, Olausson B. Craniovascular nociceptive pathways relay in the upper cervical spinal cord in the cat. Neurosci Lett. (1992) 137:203–6. doi: 10.1016/0304-3940(92)90404-U
28. Kaube H, Keay KA, Hoskin KL, Bandler R, Goadsby PJ. Expression of c-fos-like immunoreactivity in the caudal medulla and upper cervical spinal cord following stimulation of the superior sagittal sinus in the cat. Brain Res. (1993) 629:95–102. doi: 10.1016/0006-8993(93)90486-7
29. Strassman AM, Mineta Y, Vos BP. Distribution of fos-like immunoreactivity in the medullary and upper cervical dorsal horn produced by stimulation of dural blood vessels in the rat. J Neurosci. (1994) 14:3725–35. doi: 10.1523/JNEUROSCI.14-06-03725.1994
30. Piovesan EJ, Kowacs PA, Tatsui CE, Lange MC, Ribas LC, Werneck LC. Referred pain after painful stimulation of the greater occipital nerve in humans: evidence of convergence of cervical afferences on trigeminal nuclei. Cephalalgia. (2001) 21:107–9. doi: 10.1046/j.1468-2982.2001.00166.x
31. Biondi DM. Cervicogenic headache: a review of diagnostic and treatment strategies. J Am Osteopath Assoc. (2005) 105:16S–22S.15928349
32. Goadsby PJ, Zagami AS, Lambert GA. Neural processing of craniovascular pain: a synthesis of the central structures involved in migraine. Headache. (1991) 31:365–71. doi: 10.1111/j.1526-4610.1991.hed3106365.x
33. Piovesan EJ, Di Stani F, Kowacs PA, Mulinari RA, Radunz VH, Utiumi M, et al. Massaging over the greater occipital nerve reduces the intensity of migraine attacks: evidence for inhibitory trigemino-cervical convergence mechanisms. Arq Neuropsiquiatr. (2007) 65:599–604. doi: 10.1590/S0004-282X2007000400010
34. Hawkins JL, Cornelison LE, Blankenship BA, Durham PL. Vagus nerve stimulation inhibits trigeminal nociception in a rodent model of episodic migraine. Pain Rep. (2017) 2:e628. doi: 10.1097/PR9.0000000000000628
35. Kopruszinski CM, Navratilova E, Swiokla J, Dodick DW, Chessell IP, Porreca F. A novel, injury-free rodent model of vulnerability for assessment of acute and preventive therapies reveals temporal contributions of CGRP-receptor activation in migraine-like pain. Cephalalgia. (2021) 41:305–17. doi: 10.1177/0333102420959794
36. Denner AC, Vogler B, Messlinger K, De Col R. Role of transient receptor potential ankyrin 1 receptors in rodent models of meningeal nociception—experiments in vitro. Eur J Pain. (2017) 21:843–54. doi: 10.1002/ejp.986
37. Nilius B, Voets T. TRP Channels: a TR(I)P through a world of multifunctional cation channels. Pflugers Arch. (2005) 451:1–10. doi: 10.1007/s00424-005-1462-y
38. Kim YS, Son JY, Kim TH, Paik SK, Dai Y, Noguchi K, et al. Expression of transient receptor potential ankyrin 1 (TRPA1) in the rat trigeminal sensory afferents and spinal dorsal horn. J Comp Neurol. (2010) 518:687–98. doi: 10.1002/cne.22238
39. Kobayashi K, Fukuoka T, Obata K, Yamanaka H, Dai Y, Tokunaga A, et al. Distinct expression of TRPM8, TRPA1, and TRPV1 mRNAs in rat primary afferent neurons with adelta/c-fibers and colocalization with trk receptors. J Comp Neurol. (2005) 493:596–606. doi: 10.1002/cne.20794
40. Stucky CL, Dubin AE, Jeske NA, Malin SA, McKemy DD, Story GM. Roles of transient receptor potential channels in pain. Brain Res Rev. (2009) 60:2–23. doi: 10.1016/j.brainresrev.2008.12.018
41. Demartini C, Tassorelli C, Zanaboni AM, Tonsi G, Francesconi O, Nativi C, et al. The role of the transient receptor potential ankyrin type-1 (TRPA1) channel in migraine pain: evaluation in an animal model. J Headache Pain. (2017) 18:94. doi: 10.1186/s10194-017-0804-4
42. Edelmayer RM, Le LN, Yan J, Wei X, Nassini R, Materazzi S, et al. Activation of TRPA1 on dural afferents: a potential mechanism of headache pain. Pain. (2012) 153:1949–58. doi: 10.1016/j.pain.2012.06.012
43. Espi-Lopez GV, Ruescas-Nicolau MA, Nova-Redondo C, Benitez-Martinez JC, Dugailly PM, Falla D. Effect of soft tissue techniques on headache impact, disability, and quality of life in migraine sufferers: a pilot study. J Altern Complement Med. (2018) 24:1099–107. doi: 10.1089/acm.2018.0048
44. Munoz-Gomez E, Ingles M, Serra-Ano P, Espi-Lopez GV. Effectiveness of a manual therapy protocol based on articulatory techniques in migraine patients. A randomized controlled trial. Musculoskelet Sci Pract. (2021) 54:102386. doi: 10.1016/j.msksp.2021.102386
45. Dixon WJ. Efficient analysis of experimental observations. Annu Rev Pharmacol Toxicol. (1980) 20:441–62. doi: 10.1146/annurev.pa.20.040180.002301
46. Green AL, Gu P, De Felice M, Dodick D, Ossipov MH, Porreca F. Increased susceptibility to cortical spreading depression in an animal model of medication-overuse headache. Cephalalgia. (2014) 34:594–604. doi: 10.1177/0333102413515344
47. Scholpp J, Schellenberg R, Moeckesch B, Banik N. Early treatment of a migraine attack while pain is still mild increases the efficacy of sumatriptan. Cephalalgia. (2004) 24:925–33. doi: 10.1111/j.1468-2982.2004.00802.x
48. Burstein R, Yamamura H, Malick A, Strassman AM. Chemical stimulation of the intracranial dura induces enhanced responses to facial stimulation in brain stem trigeminal neurons. J Neurophysiol. (1998) 79:964–82. doi: 10.1152/jn.1998.79.2.964
49. Edelmayer RM, Vanderah TW, Majuta L, Zhang ET, Fioravanti B, De Felice M, et al. Medullary pain facilitating neurons mediate allodynia in headache-related pain. Ann Neurol. (2009) 65:184–93. doi: 10.1002/ana.21537
50. Knyihar-Csillik E, Tajti J, Mohtasham S, Sari G, Vecsei L. Electrical stimulation of the gasserian ganglion induces structural alterations of calcitonin gene-related peptide-immunoreactive perivascular sensory nerve terminals in the rat cerebral dura mater: a possible model of migraine headache. Neurosci Lett. (1995) 184:189–92. doi: 10.1016/0304-3940(94)11203-U
51. Oshinsky ML, Gomonchareonsiri S. Episodic dural stimulation in awake rats: a model for recurrent headache. Headache. (2007) 47:1026–36. doi: 10.1111/j.1526-4610.2007.00871.x
52. Robert C, Bourgeais L, Arreto CD, Condes-Lara M, Noseda R, Jay T, et al. Paraventricular hypothalamic regulation of trigeminovascular mechanisms involved in headaches. J Neurosci. (2013) 33:8827–40. doi: 10.1523/JNEUROSCI.0439-13.2013
53. Burgos-Vega CC, Quigley LD, Trevisan Dos Santos G, Yan F, Asiedu M, Jacobs B, et al. Non-invasive dural stimulation in mice: a novel preclinical model of migraine. Cephalalgia. (2019) 39:123–34. doi: 10.1177/0333102418779557
54. Cerritelli F, Ginevri L, Messi G, Caprari E, Di Vincenzo M, Renzetti C, et al. Clinical effectiveness of osteopathic treatment in chronic migraine: 3-armed randomized controlled trial. Complement Ther Med. (2015) 23:149–56. doi: 10.1016/j.ctim.2015.01.011
55. Hack GD, Koritzer RT, Robinson WL, Hallgren RC, Greenman PE. Anatomic relation between the rectus capitis posterior minor muscle and the dura mater. Spine (Phila Pa 1976). (1995) 20:2484–6. doi: 10.1097/00007632-199512000-00003
56. Scali F, Marsili ES, Pontell ME. Anatomical connection between the rectus capitis posterior major and the dura mater. Spine (Phila Pa 1976). (2011) 36:E1612–1614. doi: 10.1097/BRS.0b013e31821129df
57. MacIntosh BR, Gardiner PF, McComas AJ, editors. Skeletal Muscle: Form and Function, 2nd ed. Champaign: Human Kinetics (2006).
58. Esposito G, Setoh P, Yoshida S, Kuroda KO. The calming effect of maternal carrying in different mammalian species. Front Psychol. (2015) 6:445. doi: 10.3389/fpsyg.2015.00445
59. Esposito G, Yoshida S, Ohnishi R, Tsuneoka Y, Rostagno Mdel C, Yokota S, et al. Infant calming responses during maternal carrying in humans and mice. Curr Biol. (2013) 23:739–45. doi: 10.1016/j.cub.2013.03.041
60. Yoshida S, Esposito G, Ohnishi R, Tsuneoka Y, Okabe S, Kikusui T, et al. Transport response is a filial-specific behavioral response to maternal carrying in C57BL/6 mice. Front Zool. (2013) 10:50. doi: 10.1186/1742-9994-10-50
61. De Felice M, Ossipov MH, Wang R, Lai J, Chichorro J, Meng I, et al. Triptan-induced latent sensitization: a possible basis for medication overuse headache. Ann Neurol. (2010) 67:325–37. doi: 10.1002/ana.21897
62. Edvinsson L, Grell AS, Warfvinge K. Expression of the CGRP family of neuropeptides and their receptors in the trigeminal ganglion. J Mol Neurosci. (2020) 70:930–44. doi: 10.1007/s12031-020-01493-z
63. Eftekhari S, Salvatore CA, Calamari A, Kane SA, Tajti J, Edvinsson L. Differential distribution of calcitonin gene-related peptide and its receptor components in the human trigeminal ganglion. Neuroscience. (2010) 169:683–96. doi: 10.1016/j.neuroscience.2010.05.016
64. Rees TA, Russo AF, O'Carroll SJ, Hay DL, Walker CS. CGRP And the calcitonin receptor are co-expressed in mouse, rat and human trigeminal ganglia neurons. Front Physiol. (2022) 13:860037. doi: 10.3389/fphys.2022.860037
65. Ashina M, Hansen JM, Do TP, Melo-Carrillo A, Burstein R, Moskowitz MA. Migraine and the trigeminovascular system-40 years and counting. Lancet Neurol. (2019) 18:795–804. doi: 10.1016/S1474-4422(19)30185-1
66. Biscetti L, Cresta E, Cupini LM, Calabresi P, Sarchielli P. The putative role of neuroinflammation in the complex pathophysiology of migraine: from bench to bedside. Neurobiol Dis. (2023) 180:106072. doi: 10.1016/j.nbd.2023.106072
Keywords: osteopathic manipulative treatment, migraine-like headache, allodynia, trigeminal nucleus caudalis, trigeminal ganglia, calcitonin gene-related peptide
Citation: Byrd K, Lund M, Pan Y, Chung BH, Child K, Fowler D, Burns-Martin J, Sanikommu M, Henderson H, Gregory C, Fleming RK and Xie JY (2024) Potential mechanisms for osteopathic manipulative treatment to alleviate migraine-like pain in female rats. Front. Pain Res. 5:1280589. doi: 10.3389/fpain.2024.1280589
Received: 14 September 2023; Accepted: 15 January 2024;
Published: 5 February 2024.
Edited by:
Luca Carnevali, University of Parma, ItalyReviewed by:
Christine M. Barry, Flinders University, AustraliaAynur Özge, Board Member of International Headache Society, United Kingdom
© 2024 Byrd, Lund, Pan, Chung, Child, Fowler, Burns-Martin, Sanikommu, Henderson, Gregory, Fleming and Xie. This is an open-access article distributed under the terms of the Creative Commons Attribution License (CC BY). The use, distribution or reproduction in other forums is permitted, provided the original author(s) and the copyright owner(s) are credited and that the original publication in this journal is cited, in accordance with accepted academic practice. No use, distribution or reproduction is permitted which does not comply with these terms.
*Correspondence: Jennifer Yanhua Xie jennifer.xie@nyit.edu
†These authors have contributed equally to this work